INTRODUCTION
ABSOLUTE pitch (AP) is the rare ability to identify or produce a given pitch without a reference tone. This ability is often associated with a higher level of musicianship (Levitin, 2008). This seems obvious, since only musicians can identify a tone by correct verbal labeling. However, it has been shown that even children are able to recall familiar songs on the precise pitch level that they had used or heard many times before. This type of latent AP also indicates a relevant portion of pitch memory which is involved in the recall of pitch, independent of its octave invariant chroma or physical spectrum (Jakubowski et al., 2017). Therefore, two sub-processes of AP recognition can be differentiated: perceptual structures of processing pitch chroma, and cognitive associations with a verbal label (Elmer et al., 2015; Kim & Knösche, 2017a).
During auditory perception, the human brain analyzes both time and frequency simultaneously. Since information for AP comes from sound spectrum and time processing, time- frequency analysis of musical stimuli in AP might be an essential tool. Moreover, there is psychophysical evidence that a musical tone is characterized by two distinct dimensions: Pitch height, which represents a linear dimension caused by the increase of frequencies (e.g. by transposition by an octave), and pitch chroma, based on a cyclic octave-independent dimension of recurring tone qualities, so-called Tonigkeit (Révész, 1926; Wellek, 1963). Both have distinct representations in the human auditory cortex (Warren et al., 2003) and are crucial for absolute pitch recognition. Whilst chroma determines the harmonic and melodic aspects of music, it is robust in timbre and dynamics. Although it is not yet well understood how both pitch properties interact in AP, it seems clear that chroma is highly relevant to pitch recognition ability (Korpell, 1965), when auditory perception and identification tasks focus on pitch class rather than octave position.
It is important to consider different dimensions of sound perception that account for clearly distinguishable modes of pitch perception. These depend upon which aspect of the sound is of primary focus: either tones with independent recognition of timbre and fundamental pitch, or spectral components. Thus, Schneider and Wengenroth (2009) differentiated between two types of listeners according to their aural orientation: holistic (or fundamental) and spectral listeners. It has been demonstrated that both modes are reflected by structural and functional asymmetries in Heschl's gyrus (Schneider et al., 2005a). It remains to be shown whether AP, as a special hearing ability, is influenced by the pitch perception preference, indicated by the individual pitch perception index measured in the Pitch Perception Preference Test (PPPT, Schneider & Bleeck, 2005; Schneider et al., 2005b).
However, a great deal of discourse concerning AP centers around the question of whether it is acquired through early exposure and practice, or whether it is characterized by neural predispositions (Brown et al., 2002; Levitin & Zatorre, 2003; Ward, 1999). Twin studies reveal a familial aggregation of AP (Baharloo et al., 2000), whereas others query a simple inheritance in place of an effect of environmental, epigenetic and stochastic factors contributing to the genesis of AP (Theusch & Gitschier, 2011). Therefore, the focus here is on both presumably interacting resources. A neural predisposition for acquiring AP introduces a genetic factor, whereas exposure and early musical training during a critical period (Zatorre, 2003) brings environmental aspects of learning and culture into play. Native speakers of tonal languages (e.g. Mandarin, Cantonese, Southern Min, Vietnamese etc.) who have AP support the importance of early learning, since small children can learn to correctly pronounce a pitch-accent language, and through this develop their absolute pitch memory for tone syllables (Deutsch et al., 1999; 2004). However, the nature-nurture debate continues, and suggests an interaction of both resources; even the evidence of neural correlates and detectable substrates cannot rule out the possibility that neural changes are a possible consequence of training and early exposure.
When investigating AP, one inevitably invokes AP, as well as relative pitch (RP), with the memory system (Deutsch, 1999; Levitin, 1999). Previous research has shown that AP possessors remain more stable in their high recognition rate compared to non-possessors in a long-term memory task (Rakowski & Rogowski, 2007). Similar results are reported by Siegel (1974), showing that AP possessors substantially outperform non-possessors after a 15-seconds retention interval, when the difference between two stimuli was a semitone compared to 1/10 semitone. This might be attributed to a different coding strategy in listeners that leads to different mental representations. Therefore, AP may be seen, to some extent, as a form of tonal memory and recall from musical experience.
Much research has focused on further behavioral aspects of pitch identification (Deutsch, 2013). Effects of instrumental timbre (West, Marvin & Brinkman, 2000) and key color (Miyazaki, 1988; 1989) have been identified. Differences of reaction times (RT) in pitch labeling tasks appear between black and white key notes, with responses to white-key tones being significantly faster than to black-key tones (Bermudez & Zatorre, 2009; Miyazaki, 1989). Similarly, response time for piano tones is quicker than for string tones (West, Marvin & Brinkman, 2000), which may indicate varied degrees of easiness and fluency according to instrumental timbre. Interestingly, an interaction between AP and RP in reaction times has been found; a more global processing of listening tasks, as in AP tests, may slow down the reaction time (Ziv & Radin, 2014). The obvious prevalence of AP for different pitch classes might differ with the frequency of occurrence in the music to which the listener has been exposed (Huron, 2006).
Recently, the investigation of neural mechanisms has developed a better understanding of the underlying procedures that might explain absolute pitch processing, although such studies may be more intriguing for neuroscientists rather than musical practitioners (Schlemmer, 2008). Just recently, Hou et al. (2017) reviewed the topical literature of neuroscientific research on AP, and recapitulated the scientific knowledge of neural processes involved in AP. Here, we briefly summarize the main results. For processing pitch chroma the ventral pathway plays a critical role (Kim & Knösche, 2017b). Furthermore, left-hemisphere mechanisms facilitate pitch processing (Behroozmand et al., 2014). AP possessors also show an increased functional connectivity during music listening (Loui et al., 2012b), and a hyperconnectivity in bilateral superior temporal lobe structures (Loui et al., 2011). AP musicians exhibit a significant smaller size of the right planum temporale (Keenan et al., 2001). This increased asymmetry in AP musicians may be ascribed to genetic influences (Keenan et al., 2001). Wengenroth et al. (2014) found that gray matter volume of the right Heschl's gyrus is highly correlated with AP, and the primary auditory responses localize more posteriorly in the right hemisphere. However, the accuracy of AP skills may also be related to an early exposure to cultural norms (Hedger et al., 2013).
Finally, the memory system of AP might partly relate to synesthesia because colors and visual clues may function as markers for the characteristics of pitch chroma. Therefore, synesthesia often co-occurs with AP (Glasser, 2018; Gregersen et al., 2013). Research has demonstrated that there are shared as well as distinct neural substrates between both abilities reflected by common activation patterns (Loui et al., 2012a).
In general, the large number of research studies on AP (for a survey, see Deutsch, 2013) has revealed different dimensions involved in AP, such as perceptual and cognitive development, habitual disposition, environmental and genetic factors, and the effects of neuroanatomical conditions. In the present research, other phenomenological aspects beyond neuroscientific approaches to AP will be empirically investigated, such as the stability of the hit rate when physical sound parameters are manipulated, which might influence the accuracy of pitch recognition. The following questions stimulated the current study:
- Do pure sinusoidal tones and complex sounds differ in AP possessors' perception?
- Is the spectral structure of a complex sound relevant to pitch recognition? Does it affect the accuracy of pitch recognition?
- Which psychoacoustic parameter has the strongest influence on pitch recognition?
- Is there any correlation between the beginning of formal instrumental instruction and AP performance accuracy?
- Does the individual pitch perception preference impact pitch labeling accuracy in AP possessors?
METHOD
Participants
Fifty musicians who identified themselves as AP possessors volunteered in the study. All of them were tested by a standardized measure of AP (Schneider, 2007; Wengenroth et al., 2014) in order to verify the self-evaluation. As a result, two participants were excluded because they did not fit the standard limit of 10 points on the AP scale. According to the results, all remaining 48 participants were identified as either partial (15 participants with a score of 10 to 16 points) or full AP possessors (33 participants with a score of 16 to 34 points). The partial AP possessors consisted of 14 females and only one male participant; the 33 full AP possessors were divided into 13 males and 20 females. Of the sample, 22 participants (45.8%) were professional musicians, the same number as were music students and high school pupils. Only three individuals (6.3%) identified themselves as amateurs and one did not fit in any category. Piano (62.5%) and string players (16.6%) represented most of the sample. Mean age of the cohort was 32.3 years (range = 15 to 74 years), and participants were predominantly right handed (n = 44). Only three participants were left handed, and one participant reported being ambidextrous. Fifteen participants (31.3%) indicated that AP was present in their families (parents, siblings). The majority started with formal instrumental instruction at the age of 4 to 7 years (83.3%), whereas only a small proportion (16.7%) started later between ages 8 and 13 years.
Materials
The Tallinn Test of Absolute Pitch (TTAP, Gruhn et al., 2016) was employed to measure pitch labeling abilities. This test constitutes a listening task with single tones on nominal tuning A = 440 Hz. In total, 150 stimuli were designed, consisting of 60 synthetic instrumental tones (violin, clarinet, trumpet) in different octave ranges and dynamics, and 90 electronically-manipulated sounds, each of them in three different octave ranges (low: 100 to 400 Hz; middle: 500 to 2000 Hz; high: 2000 to 10000 Hz). The three synthetic instrumental timbres were selected because of their overtone spectra. Three types of physical manipulations were chosen for the electronic sounds that modified the spectral characteristics: 30 pure tones, 30 sounds with missing fundamentals, and 30 slightly detuned stimuli. All sounds with missing fundamentals consisted of no more than 30 partials (or less in a higher octave range) using sawtooth waveforms. All detuned sounds were composed of completely randomized partial tunings in a +/–50 cents range (quarter-tone up or down). 2 The 150 test items were presented in random order. To control effects of timbre or references made to the previous item, a tonal distortion interrupted the sequential order (for design see Figure 1).
Additionally, the Quantitative Absolute Pitch Test (Wengenroth et al., 2014) was used as a reference tool. The test was specifically designed for a quantification of the degree of AP perception as partial and full AP, and consisted of 28 equally tempered (relative to standard pitch A = 440 Hz) sampled instrumental test tones (piano, guitar, violin, organ, woodwind, brass, and voice), and six pure tones that were presented for 100 ms each in low-, middle-, and high-frequency ranges (32–138, 175–625, and 1000–2000 Hz, respectively). Different instrumental test tones have been chosen to address the possibility that AP abilities may be influenced by timbre or register. To rule out any RP-associated interval recognition, a possible recall of the last test tone was extinguished by intermittent interference stimuli without any harmonic relation to standard pitch. Five non-equally tempered sequential instrumental tones resembling and contorting the previous test tone were presented for 500 ms each followed by 18 seconds of glissando-like, continuously distorted music pieces. Only chroma independent of the octave-position was tested. For correct tones 1 point was accredited, and for semitone errors 0.5 point, resulting in a maximum score of 34 points.
The Pitch Perception Preference Test (PPPT, Schneider & Bleeck, 2005; Schneider et al., 2005b) was administered to investigate individual differences in sound perception that correlate with neuroanatomical and functional markers of the auditory brain areas. A traditional psychometric test paradigm was based on tone pairs of harmonically complex tones. Participants were asked to identify the dominant direction of pitch shift in a sample set of 144 tone pairs. The spectral components of each tone pair were composed of eight different harmonic numbers (ranging from two to 15), six different highest component frequencies (294, 523, 932, 1661, 2960 and 5274 Hz), and three different numbers of components (two, three and four). Importantly, the highest component frequency was kept constant between tones of one pair to ensure timbre consistency. For each participant, a psychometric asymmetry coefficient was derived by recording the number of holistic and spectral classifications, and computing an index of sound perception preference ranging gradually from d = – 1 .0 to d = +1.0. Subjects were categorized as holistic listeners (d < 0) who dominantly perceive the missing fundamental; as spectral listeners (d > 0) who dominantly perceive the physically present harmonics and are less capable of hearing a dominant missing fundamental; or as intermediate listeners (d ~ 0), who could perceive both the holistic and spectral cues depending on the frequency range. In some cases, intermediate listeners perceived stimuli ambiguously in the form of a conflicting pitch.
Finally, individual data were collected from two questionnaires; the pre-test questionnaire collected personal data (age, education, start of instrumental instruction, handedness, self-evaluation of the degree of AP), and a post-test questionnaire collected information about the test procedure, such as subjectively ratings of test difficulty, and the applied strategy for pitch detection.
Procedure
All participants signed a consent form informing them about the goals of the study. They agreed to participate in two sessions: A preliminary test session with the Schneider AP Test as a confirmation of AP, the Preference Test (PPPT), and the pre-test questionnaire; this was followed by the actual test session, where the Tallinn Test of Absolute Pitch (TTAP) was administered together with the post-test questionnaire.
All listening tests were given as group sessions in a quiet seminar room of the Music Academy. 3 Participants sat at tables with paper and pencil and listened to the test items through reference monitoring headphones (Beyerdynamic DT 770 Pro). After a general introduction to the procedure, three practice items confirmed the understanding of the test sequence. During the task, the test items were administered in blocks of seven items, followed by a brief musical example that was distorted by glissandi between different tonalities; this was to distract attention and confuse any tonal orientation. The participants were asked to fill the letter name (only chroma, one out of 12, without accounting for enharmonic changes) onto an answer sheet as quickly as possible (Figure 1).
The data were analyzed statistically using SPSS V.23. Background information was taken from the questionnaires and examined in relation to the behavioral data.
RESULTS
AP performance with manipulated stimuli
The total scores of TTAP significantly correlated with the Schneider AP test (r = .894, p < .01) which validates the data based on TTAP. There is also a stronger relationship between TTAP and mean accuracy for items with the manipulated sound structure of missing fundamentals (r = -.965, p < .01; see Figure 2).
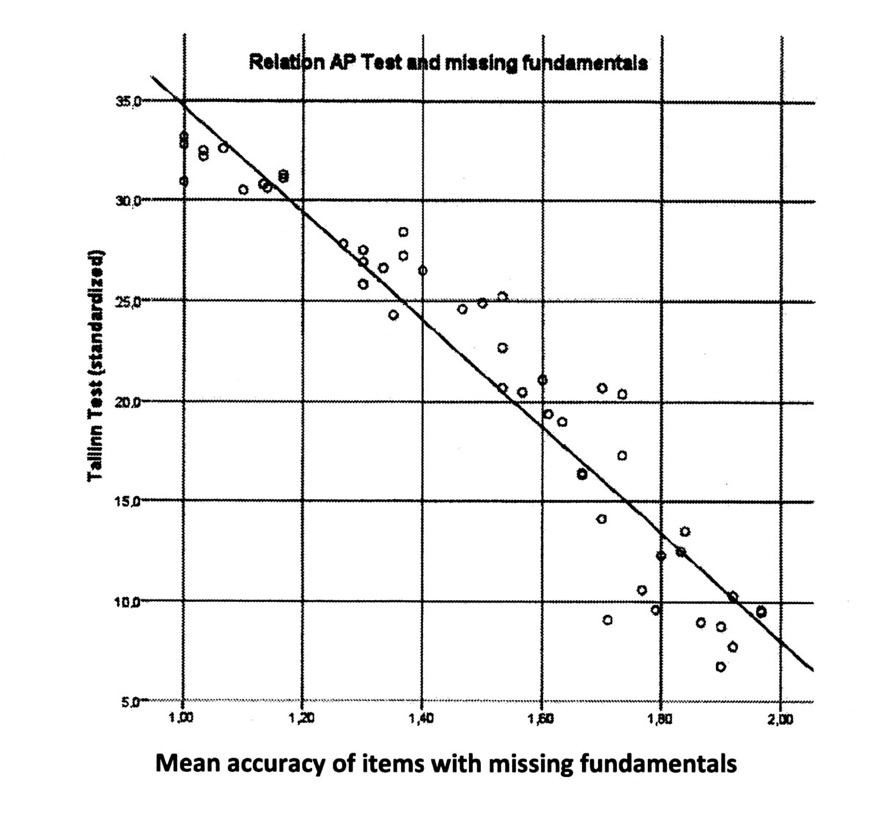
Fig. 2: Significant linear correlation of TTAP with the means of items with missing fundamentals; the higher the TTAP score, the better and more accurate a response (i.e. closer to 1, indicating a correct hit).
A comparison of the two types of AP possessors (full and partial) displays a clear differentiation between the mean scores of full AP possessors and those with partial AP (Figure 3). They score significantly lower than full AP possessors (p < .05), who responded with more correct answers. This indicates a stronger impact of psycho-acoustic parameters on the decrease of pitch recognition accuracy for partial AP possessors.
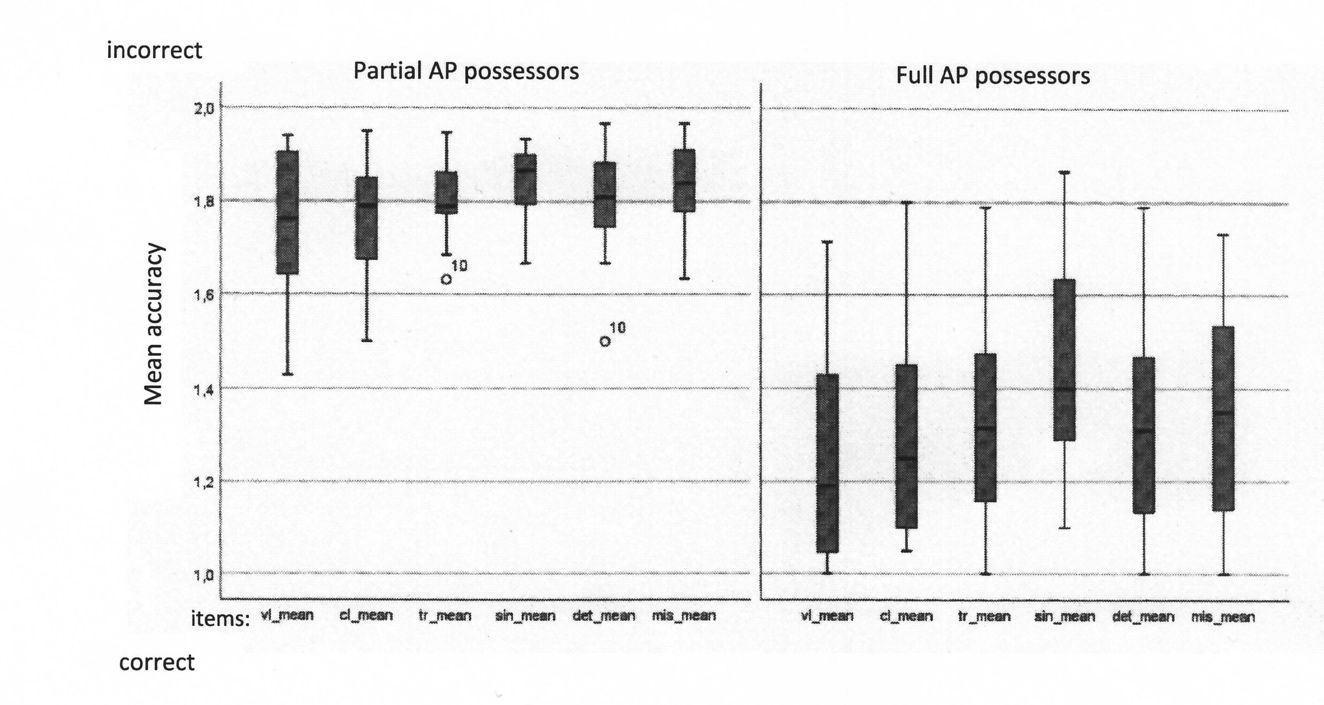
Fig. 3: Mean scores of partial (left) and full (right) AP possessors. The y-axis indicates the means between 1 (correct) and 2 (incorrect). Values indicate the means for violin (vl), clarinet (cl), trumpet (tr), sine waves (sin), detuned (det) and missing fundamental (mis) sounds.
With respect to the absolute hit rate, there is a clear decrease of correct hits from violin (1.65), through to the clarinet (1.74), trumpet (1.83) detuned (1.88), missing fundamental (1.97), and sine wave stimuli (2.06). All means are significantly different (p < .05), except between violin and clarinet stimuli, and between trumpet and detuned stimuli (Table 1).
Stimuli | Violin | Clarinet | Trumpet | Detuned | Missing f. | Sinus |
---|---|---|---|---|---|---|
N | 48 | 48 | 48 | 48 | 48 | 48 |
Mean | 1.65 | 1.74 | 1.83 | 1.88 | 1.97 | 2.06 |
Median | 1.50 | 1.57 | 1.60 | 1.75 | 1.89 | 1.97 |
SD | .59395 | .59535 | .69825 | .67321 | .69063 | .63947 |
Note: The scale refers to the degree of deviation from the correct answer: 1 = correct, 2 = error of a semitone up or down, 3 = error of two semitones up or down, and 4 = error of more than two semitones.
Pure tones versus instrumental sounds
The hit rate also differed significantly between instrumental items and acoustically manipulated items. In general, pure tones were recognized less accurately. Figure 4 shows the differentiation between violin tones and pure tones (p = .003); whereas violin tones result in the most correct hits, pure tones demonstrate a skewed distribution of answers, with higher frequencies of incorrect answers.
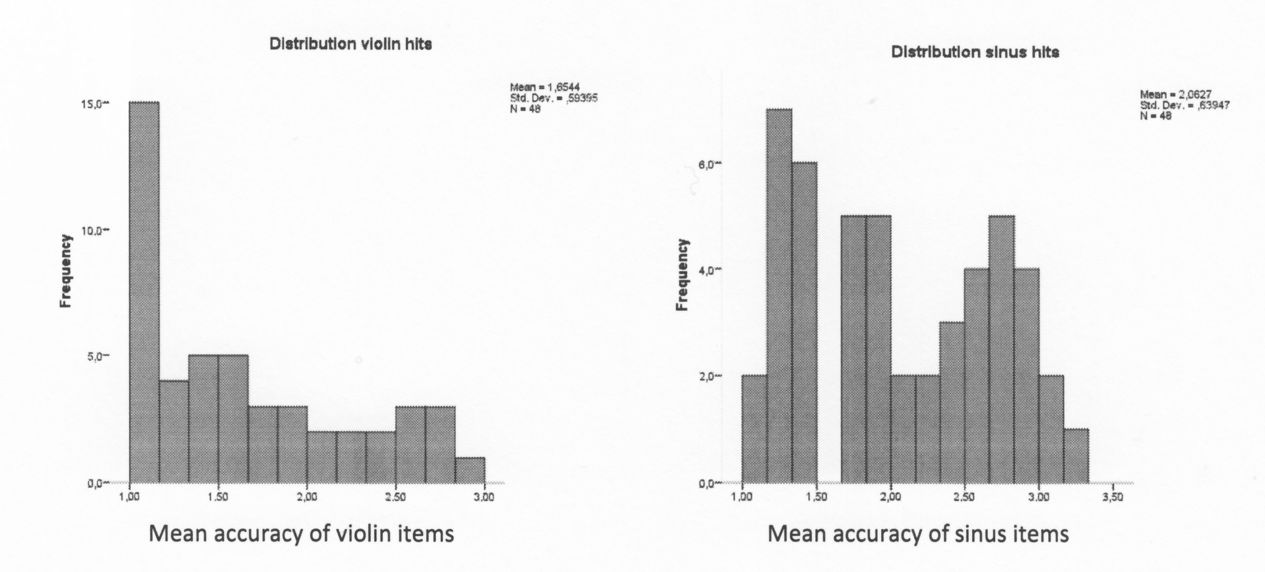
Fig. 4: Distribution of hits for violin and pure sine tones. The answers are coded according to the distance of correct hits (1 = correct; 2 = semitone deviation; 3 = whole tone deviation; 4 = more than a whole tone off target).
The Wilcoxon's signed-rank test for non-parametric distribution confirms the differences between instrumental and sine tones (violin p < .001; clarinet p < .005). A paired t-test of all correct and false responses revealed a gradual increase of false recognitions from instrumental tones to pure sine tones with an inversion of the correct – false ratio (Figure 5).
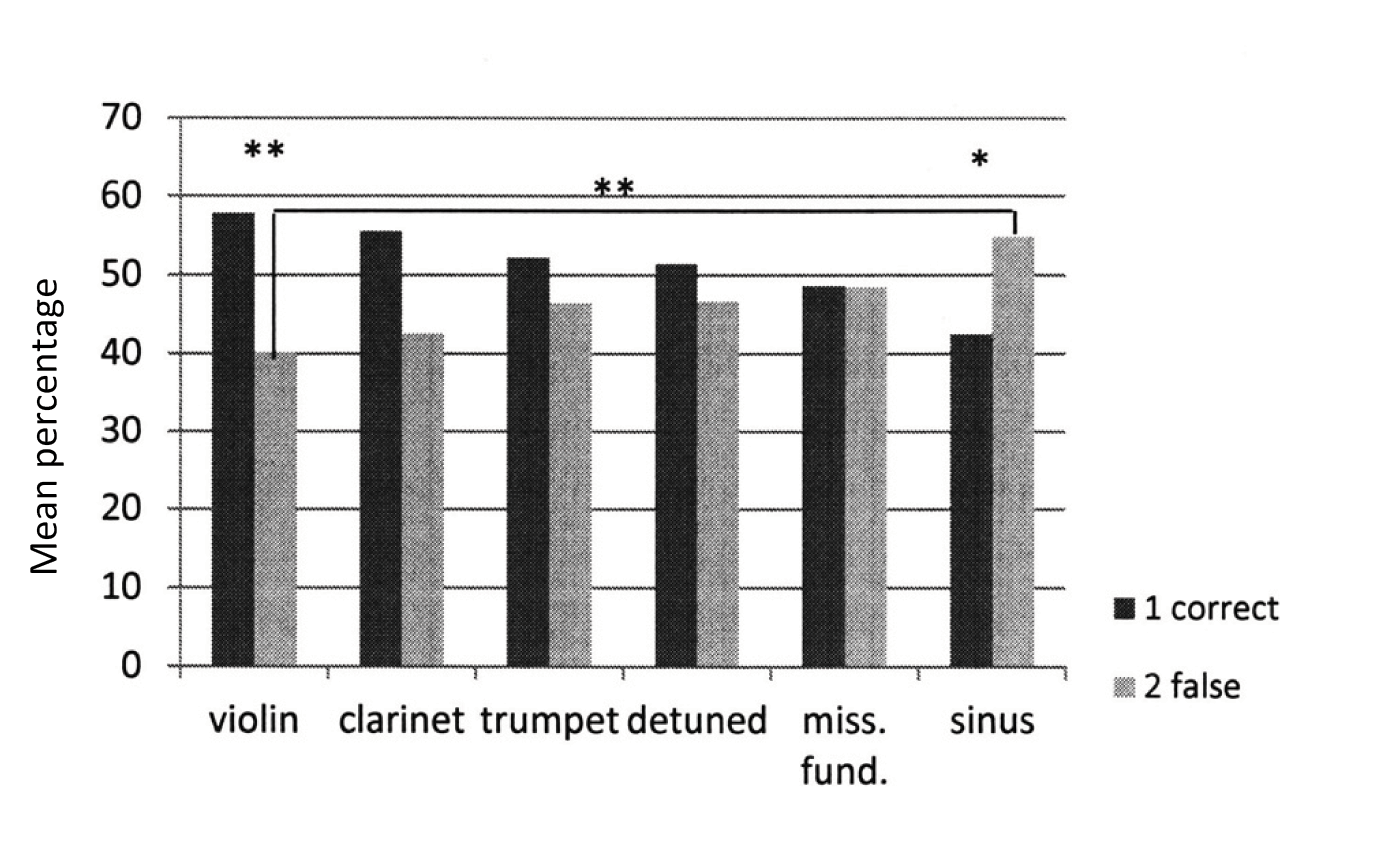
Fig. 5: The mean percentage of correct and false recognitions reveals a decrease of correct hits and, in contrast, an increase of false answers with the highest percentage in pure sine tones. The difference between errors for violin and sine tones is highly significant (significance levels: * < .05; ** < .01).
AP performance of pianists versus melodic instrument players
The differences between instrumental and acoustically-manipulated stimuli remains stable when separating the hits of pianists (30) from those of melodic instrument players (nine strings, two woodwinds, four vocals). Interestingly, melodic instrument players respond with higher hit rates that are significantly different from pianists, except for mistuned stimuli. However, the decrease in performance when moving from violin through to sine waves is more pronounced in melodic instrument players, whereas pianists show a more stable pitch detection rate, which is significantly different only for pure sine tones (p = .01). Regarding labelling inaccuracies, both groups confirm the findings from correct hits: the increase of errors is more pronounced in melodic instrument players compared to pianists (see Figure 6).
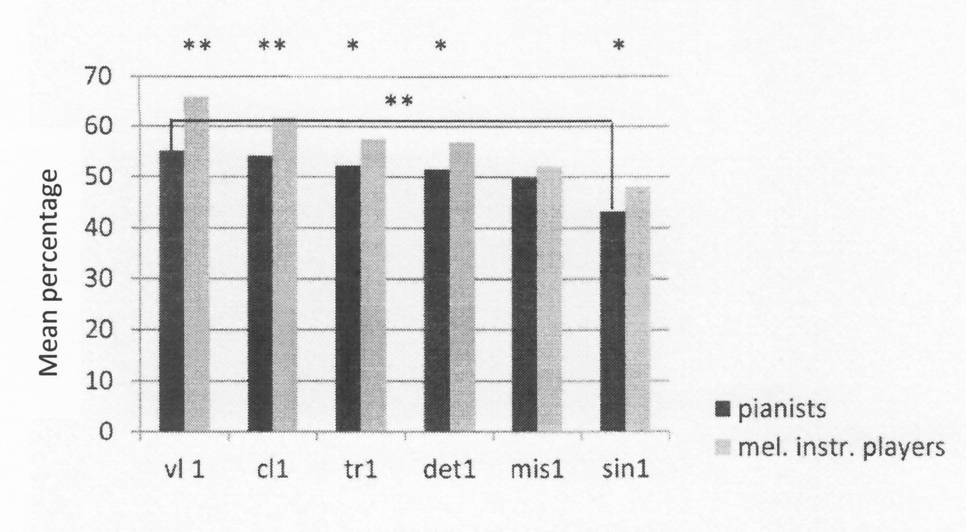
Fig. 6: Percentage of correct hits for pianists vs melodic instrument players for all items (violin, clarinet, trumpet, detuned, missing fundamental, pure sine tone) with significance levels (* < .05; ** < .01).
By looking at participants who have full AP (coded 2) it appears that there are no statistically significant differences in the hit rate among instrumental sound items, whereas significant differences exist between violin and all acoustically manipulated sounds (sine, detuned, missing fundamental), as well as between clarinet and sine waves and detuned hits (Table 2).
Pairs | t | Sig (2-tailed) |
---|---|---|
vl 2 – sin 2 | -3.279 | .004** |
vl 2 – det 2 | -2.448 | .024* |
vl 2 – mis 2 | -3.373 | .003** |
cl 2 – sin 2 | -2.168 | .043* |
cl 2 – det 2 | -2.106 | .049* |
cl 2 – mis 2 | -1.639 | .118 n.s. |
tr 2 – sin 2 | -1.441 | .167 n.s. |
tr 2 – det 2 | -1.151 | .265 n.s. |
tr 2 – mis 2 | -.618 | .545 n.s |
Effects on AP performance
In addition, we also looked for further effects on AP performance. A univariate ANOVA with TTAP score as the dependent variable, and start of instrumental instruction (age) and educational status (academic degrees) as fixed factors, exhibits a significant main effect of an early start of instruction on the TTAP score (F (9, 27) = 3.30, p = .008); however, no effect arises for the educational status on TTAP score (F (4, 27) = 2.25, p = .09). Furthermore, the occurrence of the type of AP (partial or full) strongly correlates with the TTAP score (Spearman's rho r = .793, p < .01).
The Pitch Perception Preference Test (Schneider & Bleeck, 2005; Schneider et al., 2005b) enlightens characteristic modes of pitch processing, depending on the focus on fundamentals (holistic listeners) or the overtone spectrum (spectral listeners). Data from an extended data-pool demonstrate that AP musicians have either a strong holistic or a pronounced spectral pitch perception, whereas a balanced or intermediate perception mode appears less frequently. Therefore, no specific dominance can be assigned to AP possessors. This might explain why individual perception indices from Schneider's PPPT do not show any significant effect on AP scores. Even regarding the items with missing fundamentals (which spectral listeners usually perceive weaker compared to the spectral pitches arising from harmonics), both types are not differentiated by their recognition scores in the respective TTAP section in terms of their means. However, two thirds of pure spectral listeners had difficulties recognizing items with missing fundamentals, confirming previous research findings regarding the inability to perceive missing fundamentals (Schneider & Wengenroth, 2009).
The overrepresentation of holistic listeners in our sample, however, impedes differentiated statements in this regard. Strong holistic sound perception (-1 to -0.5) dominated the individual perception modes, which might be because most participants were pianists (62.5%). Overall, 21 of a total of 30 pianists (70%) were pure holistic listeners (see Figure 7).
Black and white keys
When considering hits and errors in terms of black and white keys, it is striking that 53% of correct hits refer to white keys, whereas only 42 % refer to black keys. The better rates for white-key notes are in line with corresponding research findings about differences in reaction time (Miyazaki, 1988). This finding might be a consequence of the dominance of Western music, where white keys prevail in ordinary musical practice. Consequently, the correct recognition is linked with the recall of more prominent or dominant pitches, supporting an impact of cultural exposure.
Other visual and corporeal associations
If memory plays an important role, it may be assumed that certain associations are linked with AP. We found 26% of the sample indicated associations with visual clues that might refer to a connection with synesthesia; 20% indicated body sensations as a main association, whereas the majority (62%) referred to "other" associations such as instrumental fingering (motor and body sensation), or the laryngeal position. However, most participants claimed no awareness of any specific strategy to memorize and recognize sound.
Aspects of gender and handedness were not addressed due to the imbalanced gender-related structure of the sample, and the prevalence of right-handed participants.
DISCUSSION
The study relates the obvious phenomenological aspects of AP to the psychophysical scaffolding of pitch recognition in AP possessors. In view of this relation, AP constitutes a musical skill that results from genetic factors, and from environmental impact that originates from learning and practice. AP, then, represents a special form of a musical trait that seems to come naturally (one has it or not), but it can also, at least partly, be acquired. The first (one has it) is due to genetics, the latter (partly acquired) to memory and training. Both may occur independently, but they can also interact. The current, significant main effect of an early start in instrumental instruction underscores the importance of an environmentally supported training effect and confirms earlier findings (Sergeant, 1969).
One can assume that the portion of AP that is acquired and memorized is more error-prone than the genetically determined ability, because the former is founded in clear neuronal correlates beyond training, acquaintance or usage and, therefore, might stabilize pitch recognition. This study aimed to show how stable pitch recognition accuracy would be in a broader sample of AP possessors.
Musicians are accustomed to the sound of natural musical instruments. However, the current study also dealt with psychoacoustic manipulations of the physical structure of a perceived pitch. Why does it make a difference to listen to a natural instrumental sound, or to sounds that are slightly detuned or characterized by a harmonic spectrum with a missing fundamental (that is, when the perceived pitch frequency is not physically present, but must be generated in the musician's mind due to the overtone spectrum of that sound)? Why is a pure tone not similarly perceived as a complex tone with its rich overtone spectrum? Musicians exhibit an expert knowledge of sounds by the experience of daily practice. They "feel" that both types of sound structures are acoustically different. Therefore, it was expected that musicians respond to varying types of stimuli in our test differently, and that varied instrumentalists process pitches differently according to the sound spectra of their familiar instruments. Here, the question was how stable pitch labeling accuracy arises under those various conditions.
The data of the test confirm that there is a clear hierarchy of accurate pitch recognition from pitches with a natural spectrum of overtones down to a pure sinusoidal sound. The individual percept of complex instrumental sounds is obviously different from other sound sources, so that manipulated sounds are less accurate in an AP recognition test than natural instrumental sounds, although all items were synthetically produced. It seems reasonable that a sound can best deploy its unique characteristics through an instrument. Moreover, the sound spectrum that is responsible for the instrumental timbre might influence pitch detection in the case of spectral listeners, but less so for fundamental pitch listeners. For fundamental pitch listeners, the pitch percept of a complex sound should be independent of the shape and intensity of the sound spectrum due to its specific focus on the fundamental pitch. In contrast, the pitch percept of spectral listeners may be influenced by the sound spectrum characteristics, due to their sensitivity to spectral pitches and formants. This is clearly reflected in the difficulty of spectral listeners to recognize the correct (fundamental) pitch of the physically manipulated tones. However, pitch chroma is strongly connected with the overtone spectrum, but independent from the formant structure that determines instrumental timbre. Consequently, any intervention of the sound structure by physical manipulation (e.g. by eliminating the fundamentals or detuning elements of the spectrum) will necessarily influence the recognition rate.
On the other hand, pure tones exhibit an astonishing failure in recognition, which confirms the findings of Lee and Lee (2010) and Glasser (2018). There are several reasons for this: first, the weaker recognition ability of sinusoidal tones might be due to the fact that the pitch height of pure tones are less pronounced in higher and lower pitch ranges (Terhardt, 1998). Furthermore, the detailed analyses of the PPP test (Schneider et al., 2005b) demonstrate that the strength of the fundamental pitch percept correlates with the average number of harmonics that are physically present in a missing fundamental sound. In the case of an incomplete complex sound with only two present harmonics, spectral listeners perceive just the spectral pitches corresponding to the frequencies of the two harmonics. In the case of three or more harmonics, the spectral listeners show a slow, systematic increase in strength of fundamental pitch perception, even though the fundamental pitch is still missing. For fundamental listeners, the fundamental pitch percept is always present independent of changes in the sound spectrum, but also strengthens with an increasing number of harmonics (Schneider, Sluming et al., 2005b). Considering these observations, it is not surprising that sinusoidal tones are correctly recognized less often than natural complex tones, although they clearly indicate pitch height in terms of frequency. Therefore, spectral chroma seems to be a prerequisite for pitch detection, independent of individual differences in pitch perception preference.
Furthermore, in our test it seems remarkable that melodic instrument players always performed at a higher level compared with pianists (see Figure 6). However, pianists are more stable in their correct pitch detection rate, despite a striking drop of correct detection in pure tones. These inverse observations for pianists and melodic instrument players may be explained by the fact that pianists on average show a considerably stronger fundamental pitch percept compared to melodic instrument players and singers (Schneider et al., 2005a). Nevertheless, their higher spectral pitch awareness may have an influence on the performance accuracy of the octave-independent pitch chroma perception. Overall, the strength of AP perception might be considerably enhanced if pitch chroma is not only derived from fundamental pitch, but also from an octave-shifted fundamental pitch with the same chroma. Indeed, the recognition of octave-shifted pitches occurs more frequently in spectral listeners (Schneider et al., 2005b; Schneider & Wengenroth, 2009). As melodic instrument players show a higher rate of spectral pitch perception compared to pianists, they should consequently have a higher AP accuracy compared to fundamental pitch listeners, who derive the AP information predominantly from the non-octavated fundamental pitch. The influence of octave ambiguity on pitch labeling accuracy may be underestimated so far.
It seems plausible that error-proneness is higher when more memory is involved. If recognition is derived from a neurally determined ability, it can be expected that the accuracy of pitch recognition is higher. Therefore, further research might focus on the relation of neural correlates with empirical accuracy of pitch recognition in AP tests, and investigate to what extent neural correlates predict the error-rate or pitch detection accuracy.
The missing effects of the perception indices on AP scores was unexpected, but might be due to the uneven distribution (see Figure 7) of listening modes and instrumental orientation of the participants. The majority consisted of designated holistic listeners (81%) with a predominance of piano players that is purported to be a preferred instrument of this type of sound perception (Schneider et al., 2005a). Both listening modes (holistic vs spectral) are neurally determined and do not exhibit training effects. The measurable perception index refers to a mental disposition of sound processing that is based on neural traits that obviously develop independently from the neural conditions for AP abilities. In contrast, AP has, beyond the neural correlates, a strong association with memory that is irrelevant for the perception index. Therefore, it is reasonable to suggest that perception indices do not qualify as predictors of AP competence.
The apparent differences between black and white keys in pitch recognition shed light on the importance of exposure to music, and the practical acquisition of percepts developed by playing a musical instrument. The superiority of white keys compared to black keys mirrors the statistical dominance of tones without alterations in traditional classical music. This underpins once more the importance of cultural imprints beyond genetics. The Western tonal system forms European instruments as well as the mental representation of their sounds. Consequently, pitches of a Western tonal system are more stably represented than other detuned pitches. To mark the traits of those pitches, the ear needs information from the sound structure; the more this is modified, or even violated, the less accurate pitch detection may be.
An interesting result was found regarding visual clues during pitch recognition in the AP test. Although we cannot assert strong evidence for synesthesia, the small number of participants who refer to visual clues may nevertheless be an indication of a possible involvement of synesthetic effects in pitch recognition, supporting the idea that synesthesia and AP represent "two sides of the same coin" (Loui et al., 2012a). This is confirmed by a study that demonstrates that most AP cases in the sample met the diagnostic criteria for synesthesia (Glasser, 2018). In fact, with regard to a multisensory integration, one can assume that tonal and visual modes of processing are connected in AP (Loui et al., 2012a; Spence & Soto-Faraco, 2010), but this requires further investigation.
From this study, it can be concluded that pitch recognition in AP possessors is not just a distinct ability based on simple mechanisms. Instead, it is based on a mutual interaction of genetic and environmental, mental and memory dependent factors that influence the reliability of pitch labeling ability even in AP possessors.
ACKNOWLEDGMENTS
This article was copyedited by Tanshuree Agrawal and layout edited by Diana Kayser.
NOTES
- Correspondence can be addressed to: Prof. Dr. Wilfried Gruhn, Lärchenstr. 5, D-79256 Buchenbach, mail@wgruhn.de
Return to Text - All sound items were electronically designed and produced in the Electronic Music Studio at the Estonian Academy of Music by Tammo Sumera.
Return to Text - Test sessions were organized and administered by Reet Ristmägi, Meeta Morozov and Karl Joosep Sinisalu from the Estonian Academy of Music and Theatre, one test session was performed at Heino Eller Music High School in Tartu, Estonia.
Return to Text
REFERENCES
- Baharloo, S., Service, S.K., Risch, N., Gitschier, J., & Freimer, N.B. (2000). Familial aggregation of absolute pitch. American Journal of Human Genetics, 67(3), 755-758. https://doi.org/10.1086/303057
- Behroozmand, R., Ibrahim, N., Korzyukov, O., Robin, D.A., & Larson, C.R. (2014). Left-hemisphere activation is associated with enhanced vocal pitch error detection in musicians with absolute pitch. Brain and Cognition, 84(1), 97 - 108. https://doi.org/10.1016/j.bandc.2013.11.007
- Bermudez, P., & Zatorre, R.J. (2009). A distribution of absolute pitch ability as revealed by computerized testing. Music Perception, 27(2), 89-101. https://doi.org/10.1525/mp.2009.27.2.89
- Brown, W.A., Sachs, H., Cammuso, K., & Folstein, S.E. (2002). Early music training and absolute pitch. Music Perception, 19, 595-597. https://doi.org/10.1525/mp.2002.19.4.595
- Deutsch, D. (Ed.). (1999). The psychology of music (second edition). San Diego: Academic Press.
- Deutsch, D. (2013). Absolute pitch. In D. Deutsch (Ed.), The psychology of music, 3rd. edition (pp. 141-182). San Diego: Academic Press. https://doi.org/10.1016/B978-0-12-381460-9.00005-5
- Deutsch, D., Henthorn, T., & Dolson, M. (1999). Absolute pitch is demonstrated in speakers of tone languages. Journal of the Acoustical Society of America, 106, 2267. https://doi.org/10.1121/1.427738
- Deutsch, D., Henthorn, T., & Dolson, M. (2004). Absolute pitch, speech, and tone language: some experiments and a proposed framework. Music Perception, 21(3), 339 - 356. https://doi.org/10.1525/mp.2004.21.3.339
- Elmer, S., Rogenmoser, L., Kuhnis, J., & Jäncke, L. (2015). Bridging the gap between perceptual and cognitive perspectives on absolute pitch. Journal of Neuroscience, 35, 366 - 371. https://doi.org/10.1523/JNEUROSCI.3009-14.2015
- Glasser, S. (2018). The impact of synaesthesia and absolute pitch on musical development. Doctor of Philosophy Thesis, The University of Melbourne.
- Gregersen, P.K., Kowalsky, E., Lee, A., Baron-Cohen, S., Fisher, S.E., Asher, J.E., Ballard, D., Freudenberg, J., & Li, W. (2013). Absolute pitch exhibits phenotypic and genetic overlap with synesthesia. Human Molecular Genetics, 22(10), 2097 - 2104. https://doi.org/10.1093/hmg/ddt059
- Gruhn, W., Ristmägi, R., & Kiili, K. (2016). Tallinn Test of Absolute Pitch (TTAP). unpublished.
- Hedger, S.C., Heald, S.L., & Nusbaum, H.C. (2013). Absolute pitch may not be so absolute. Psychological Science, 24(8), 1496 - 1502. https://doi.org/10.1177/0956797612473310
- Hou, J., Chen, A.C., Song, B., Sun, C., & Beauchaine, T.P. (2017). Neural correlates of absolute pitch: A review. Musicae Scientiae, 21(3), 287-302. https://doi.org/10.1177/1029864916662903
- Huron, D. (2006). Sweet anticipation. Music and the psychology of expectation. Cambridge MA: MIT Press. https://doi.org/10.7551/mitpress/6575.001.0001
- Jakubowski, K., Müllensiefen, D., & Stewart, L. (2017). A developmental study of latent absolute pitch memory. Quarterly Journal of Experimental Psychology, 70(3), 434 - 443. https://doi.org/10.1080/17470218.2015.1131726
- Keenan, J.P., Thangaraj, V., Halpern, A.R., & Schlaug, G. (2001). Absolute pitch and planum temporale. Neuroimage, 14(6), 1402 - 1408. https://doi.org/10.1006/nimg.2001.0925
- Kim, S.G., & Knösche, T.R. (2017a). On the perceptual subprocess of absolute pitch. Frontiers in Neuroscience, 11: 557. https://doi.org/10.3389/fnins.2017.00557
- Kim, S.G., & Knösche, T.R. (2017b). Resting state functional connectivity of the ventral auditory pathway in musicians with absolute pitch. Human Brain Mapping, 38(8), 3899 - 3916. https://doi.org/10.1002/hbm.23637
- Korpell, H.S. (1965). On the mechanism of tonal chroma in absolute pitch. American Journal of Psychology, 78(2), 298 - 300. https://doi.org/10.2307/1420505
- Lee, C.-Y., & Lee, Y.-F. (2010). Perception of musical pitch and lexical tones by Mandarin-speaking musicians. Journal of the Acoustical Society of America, 127, 481-490. https://doi.org/10.1121/1.3266683
- Levitin, D.J. (1999). Absolute pitch: self-reference and human memory. International Journal of Computing Anticipatory Systems, 4, 255 - 266.
- Levitin, D.J. (2008). Absolute pitch: both a curse and a blessing. In M. Clockars & M. Peltomaa (Eds.), Music meets medicine. Proceedings of the Signe and Ane Gyllenberg Foundation (pp. 124 - 132). Helsinki: Signe and Ane Gyllenberg Foundation.
- Levitin, D.J., & Zatorre, R.J. (2003). On the nature of early music training and absolute pitch: A reply to Brown, Sachs, Cammuso, and Folstein. Music Perception, 21(1), 105-110. https://doi.org/10.1525/mp.2003.21.1.105
- Loui, P., Li, H.C., Hohmann, A., & Schlaug, G. (2011). Enhanced cortical connectivity in absolute pith musicians: a model for local hyperconnectivity. Journal of Cognitive Neuroscience, 23(4), 1015-1026. https://doi.org/10.1162/jocn.2010.21500
- Loui, P., Zamm, A., & Schlaug, G. (2012a). Absolute pitch and synesthesia: two sides of the same coin? Shared and distinct neural substrates of music listening. ICMPC (publ. in PMC 2013), 618 - 623.
- Loui, P., Zamm, A., & Schlaug, G. (2012b). Enhanced functional networks in absolute pitch. Neuroimage, 63(2), 632 - 640. https://doi.org/10.1016/j.neuroimage.2012.07.030
- Miyazaki, K. (1988). Musical pitch identification by absolute pitch possessors. Perception and Psychophysics, 44(6), 501-512. https://doi.org/10.3758/BF03207484
- Miyazaki, K. (1989). Absolute pitch identification: effects of timbre and pitch region. Music Perception, 7(1), 1-14. https://doi.org/10.2307/40285445
- Rakowski, A., & Rogowski, P. (2007). Experiments on long-tern and short-term memory for pitch in musicians. Archives of Acoustics, 32, 815-826.
- Révész, G. (1926). Zur Geschichte der Zweikomponententheorie der Tonhöhe. Zeitschrift für Psychologie, 99, 325 - 356.
- Schlemmer, K.B. (2008). Absolutes Hören. In H. Bruhn, R. Kopiez, & A.C. Lehmann (Eds.), Musikpsychologie. Das neue Handbuch (pp. 490 - 498). Reinbel: Rowohlt.
- Schneider, P. (2007). Test zur graduellen Erfassung des absoluten Gehörs. Heidelberg: Universitätsklinikum.
- Schneider, P., & Bleeck, S. (2005). Test zur Grundton- und Obertonerkennung (Pitch Perception Preference Test). unpubl.
- Schneider, P., Sluming, V., Roberts, N., Bleeck, S., & Rupp, A. (2005a). Structural, functional, and perceptual differences in Heschl's gyrus and musical instrument preference. Annals of the New York Academy of Sciences, 1060, 387 - 394. https://doi.org/10.1196/annals.1360.033
- Schneider, P., Sluming, V., Roberts, N., Scherg, M., Goebel, R., Specht, H.J., Dosch, H.G., Bleeck, S., Stippich, C., & Rupp, A. (2005b). Structural and functional asymmetry of lateral Heschl's gyrus reflects pitch perception preference. Nature Neuroscience, 8(9), 1241 - 1247. https://doi.org/10.1038/nn1530
- Schneider, P., & Wengenroth, M. (2009). The neural basis of individual holistic and spectral sound perception. Contemporary Music Review, 28(3), 315 - 328. https://doi.org/10.1080/07494460903404402
- Sergeant, D.C. (1969). Experimental investigation of absolute pitch. Journal of Research in Music Education, 17, 135 - 143. https://doi.org/10.2307/3344200
- Siegel, J.A. (1974). Sensory and verbal coding strategies in subjects with absolute pitch. Journal of Experimental Psychology, 103, 37-44. https://doi.org/10.1037/h0036844
- Spence, C., & Soto-Faraco, S. (2010). Auditory perception: interactions with vision. In C.J. Plack (Ed.), Oxford Handbook of Auditory Science: Hearing (pp. 271 - 296). Oxford: Oxford University Press. https://doi.org/10.1093/oxfordhb/9780199233557.013.0012
- Terhardt, E. (1998). Akustische Kommunikation. Grundlagen mit Hörbeispielen. Berlin et al.: Springer. https://doi.org/10.1007/978-3-642-58733-7
- Theusch, E., & Gitschier, J. (2011). Absolute pitch twin study and segregation analysis. Twin Research and Human Genetics, 14(2), 173-178. https://doi.org/10.1375/twin.14.2.173
- Ward, W.D. (1999). Absolute pitch. In D. Deutsch (Ed.), The psychology of music (second edition) (pp. 265 - 298). San Diego: Academic Press. https://doi.org/10.1016/B978-012213564-4/50009-3
- Warren, J.D., Uppenkamp, S., Patterson, R.D., & Griffith, T.D. (2003). Separating pitch chroma and pitch height in the human brain. Proceedings of the National Academy of Sciences in the USA, 100(17), 10038 - 10042. https://doi.org/10.1073/pnas.1730682100
- Wellek, A. (1963). Musikpsychologie und Musikästhetik. Frankfurt am Main: Akad. Verlagsgesellschaft.
- Wengenroth, M., Blatow, M., Heinecke, A., Reinhardt, J., Stippich, C., Hofmann, E., & Schneider, P. (2014). Increased volume and function of right auditory cortex as a marker for absolute pitch. Cerebral Cortex, 24(5), 1127 - 1137. https://doi.org/10.1093/cercor/bhs391
- West Marvin, E., & Brinkman, A.R. (2000). The effect of key color and timbre on absolute pitch recognition in musical contexts. Music Perception, 18(2), 111-137. https://doi.org/10.2307/40285905
- Zatorre, R.J. (2003). Absolute pitch: a model for understanding the influence of genes and development on neural and cognitive function. Nature Neuroscience, 6, 692 - 695. https://doi.org/10.1038/nn1085
- Ziv, N., & Radin, S. (2014). Absolute and relative pitch: global versus local processing of chords. Advances in Cognitive Psychology, 10(1), 15 - 25. https://doi.org/10.5709/acp-0152-7